What is gene technology?
Genetic engineering is the process of using advanced DNA technology to modify the genetic content of an organism. Scientists can use genetic engineering to isolate genes and then combine them with the genome of the recipient DNA. The DNA created from this combination is called recombinant DNA.
The organism that contains recombinant DNA is a transgenic or a genetically modified organism (GMO), which can be as simple as bacteria or as complex as plants and animals.
Modifying the genetic makeup of organisms is a complex process that involves utilising genetic engineering techniques in multiple steps.
Genetic engineering aims to develop GMOs with more desirable outcomes. Crops, such as corn, soybeans, and cotton can be genetically modified to resist pesticides. This helps farmers have a better yield since they can use pesticides to eradicate pests without damaging the crops themselves. Bacteria can also be genetically modified. Scientists can introduce a gene that encodes a valuable protein (a drug or a hormone such as insulin) into a bacterium. By growing the new bacterial colonies in bulk, they can collect and purify the desired protein and use it for pharmaceutical or industrial purposes.
The genetic code is universal, meaning it is the same in all organisms. Therefore, the DNA is transcribed and translated within the transgenic organisms the same way it is in the donor organism, producing the same protein.
Here are some benefits of genetic engineering:
- Allows for creating more nutritious foods to eradicate malnutrition around the world.
- Disease and drought resistant plants allow for the use of fewer environmental resources.
- Increased food supply with lower cost.
- Increased shelf life of certain rootstock.
- Greater yields in farming crops for making biofuels.
- Faster growing crops and animals.
Fig. 1 - Benefits of genetic engineering
Genetic engineering is not all benefits. There are some implications and disadvantages that come with it. These include:
- The food produced from GMO animals can have less nutritional value due to a faster rate of development.
- Introducing new genetic material into nature can result in new resistant pathogens that are stronger and pose a risk to health concerns.
- There can be adverse side effects that are unknown and unexpected.
- Many companies place copyright over the GMOs they develop. It can have costly consequences for the farmers since they would have to pay greater GMO seeds.
- Abuse of genetic engineering technology and knowledge on animals and humans can lead to ethically questionable outcomes.
Fig. 2 - Drawbacks and ethical implications of genetic engineering
We can break down the process of creating recombinant DNA and transferring it successfully to create a GMO into five main steps:
- The gene that encodes the desired product (e.g., protein) must be isolated. This process involves producing DNA fragments that contain the desirable gene.
- The gene then needs to be inserted into a vector.
Vectors are carriers for delivering foreign genetic material, such as a functional gene, directly into a cell.
- The vector delivers the gene to the recipient cells. This delivery process is called transformation.
Transformation describes the cell's genetic modification via direct uptake and inclusion of foreign genetic materials from its surroundings.
- The recombinant cells that have successfully transformed and now contain the recombinant DNA need to be identified. Marker genes, such as antibiotic resistance genes, assist in this step.
- Following identification, the recombinant cells are cloned to produce a large population of transgenic cells.
Producing DNA fragments via gene technology
Identifying and isolating a specific gene that is a few hundred bases in length amongst millions of bases in eukaryotic DNA is quite challenging. There are three main techniques that scientists use to create DNA fragments:
- Using reverse transcriptase to convert mRNA (messenger RNA) to cDNA (complementary DNA).
- Using restriction endonuclease to cut the DNA molecule at a specific sequence.
- Using a gene machine to create a gene-based on a protein with a known structure and composition.
Reverse transcription
This process uses a particular enzyme called reverse transcriptase. This enzyme naturally occurs in retroviruses such as HIV, and it creates DNA strands based on mRNA molecules.
- mRNA in cells correspond to the genetic sequence, and ribosomes read them to synthesise polypeptide molecules (proteins). This process is known as translation.
- A cell that naturally produces a protein should contain large amounts of that protein's mRNA, which can be isolated. For example, the beta cells in the islets of Langerhans in the pancreas secrete insulin and should contain high levels of insulin mRNA. So, the insulin mRNA can be extracted from beta cells.
- Following extraction of the desired mRNA, they can be treated with the reverse transcriptase enzyme, which polymerises deoxyribonucleotides and creates single-stranded cDNA molecules that have a complementary base sequence to that of the mRNA.
Complementary DNA or cDNA is synthesised via the reverse transcription procedure based on a single-stranded RNA molecule such as mRNA.
- The obtained cDNA molecules are subsequently treated with DNA polymerase in the polymerase chain reaction (PCR) process. The DNA polymerase synthesises a complementary DNA strand based on the cDNA, creating a double-stranded DNA. It also replicates the DNA molecules in repeated cycles that amplifies the number of DNA fragments.
- The main advantage of the reverse transcription process in isolating genes is that the product created at the end is a cDNA that does not contain any non-coding DNA (introns). This is important for inserting genes into prokaryotes since prokaryotic systems cannot remove introns.
Restriction endonucleases
Restriction endonucleases (RE) are enzymes that are naturally part of the bacteria's defence mechanism. They act by cutting the foreign DNA molecules.
There are various types of REs with active sites complementary to specific bases nucleotide sequences. These sequences are called recognitions sites, since each RE creates incisions in the DNA specifically at these locations.
We can classify REs into two main groups based on how they cut the DNA:
- REs that incise the DNA at the exact location on both strands creates two blunt ends.
- REs that cut both DNA strands at positions a few bases apart from each other lead to the creation of staggered ends with exposed DNA bases (bases without complementary pairs). These unpaired DNA bases can join to a DNA with complementary bases. These ends are also called sticky ends since they can easily join other DNA samples with sticky ends. The recognition sites for these types of REs have a palindromic sequence, meaning they are read the same on the forwards and reverse strands.
Fig. 3 - Creating DNA fragments with sticky ends using restriction enzymes
The gene machine
The gene machine is the most modern technique where scientists create DNA fragments in a laboratory using computers and special devices.
They first identify the protein of interest and examine its constituting amino acid sequence. Then, scientists can determine the mRNA and DNA sequences that could encode that protein by using the genetic code in reverse.
The obtained DNA sequence can then be entered into the computer. The computer checks the DNA fragments for biosafety and biosecurity, ensuring that it follows the various ethical regulations and does not violate international regulations. The computer then creates a series of small single-stranded nucleotides with overlapping sequences in an automated process. These strands are called oligonucleotides and can be assembled to generate the DNA sequence of the desired gene. Lastly, the engineered DNA strand is amplified and converted to the double-stranded DNA molecule using PCR.
Oligonucleotides are polynucleotides that contain relatively small numbers of nucleotides.
The gene machine technique is accurate and can be conducted in as little as ten days. It also creates DNA strands that do not contain any non-coding regions (introns) and can be transcribed and translated by prokaryotic systems.
Table 1. Advantages and disadvantages of different gene technologies summarised.
| Advantages | Disadvantages |
Reverse transcriptase technique |
|
|
Restriction endonuclease technique |
|
|
Gene machines |
|
|
Gene Technology - Key takeaways
- Genetic engineering is the process of using advanced DNA technology to modify the genetic content of an organism.
- The organism that contains this recombinant DNA is a transgenic or a genetically modified organism (GMO), which can be as simple as bacteria or as complex as plants and animals.
- Application of GMO:
- Production of valuable proteins in bulk, such as insulin.
- Creation of pesticide- and herbicide-resistant crops.
- Five main steps of transferring recombinant DNA to create a GMO:
- Isolation of the desired gene.
- Insertion of the gene into a vector.
- Transformation.
- Identification of transformed cells.
- Cloning of the amplified cells.
- Three main techniques for creating DNA fragments:
- Reverse transcription.
- Usage of restriction endonucleases.
- Gene machine.
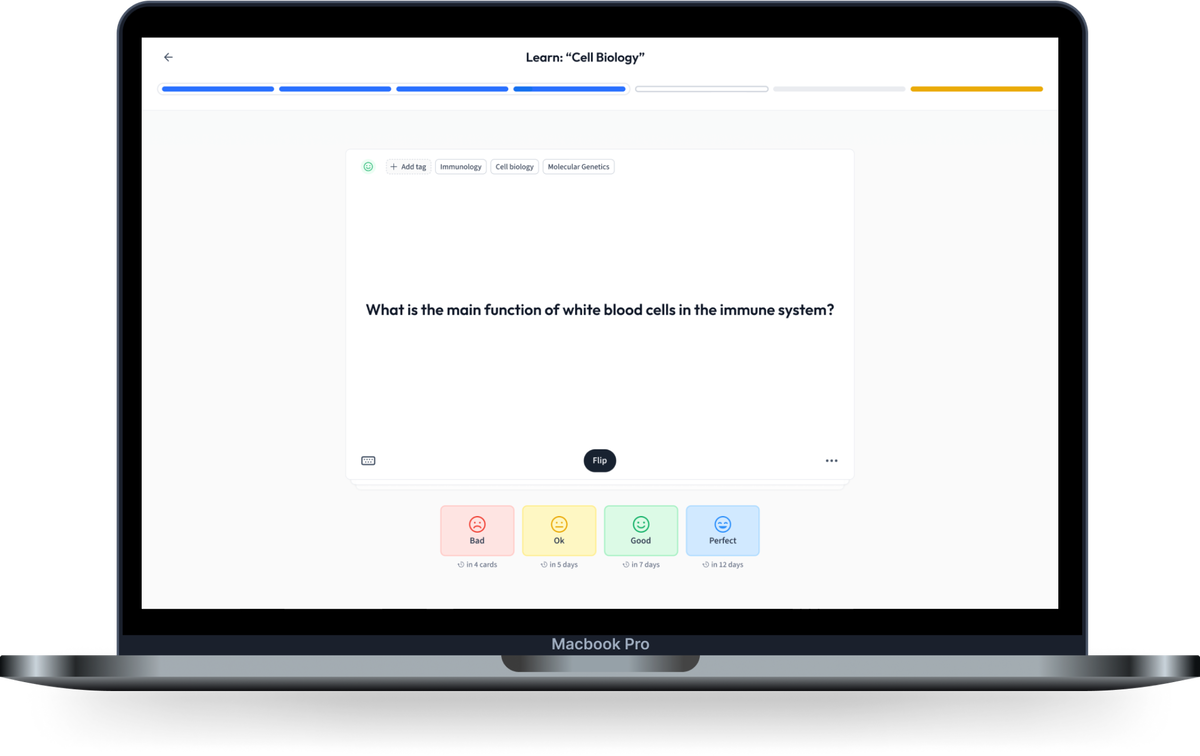
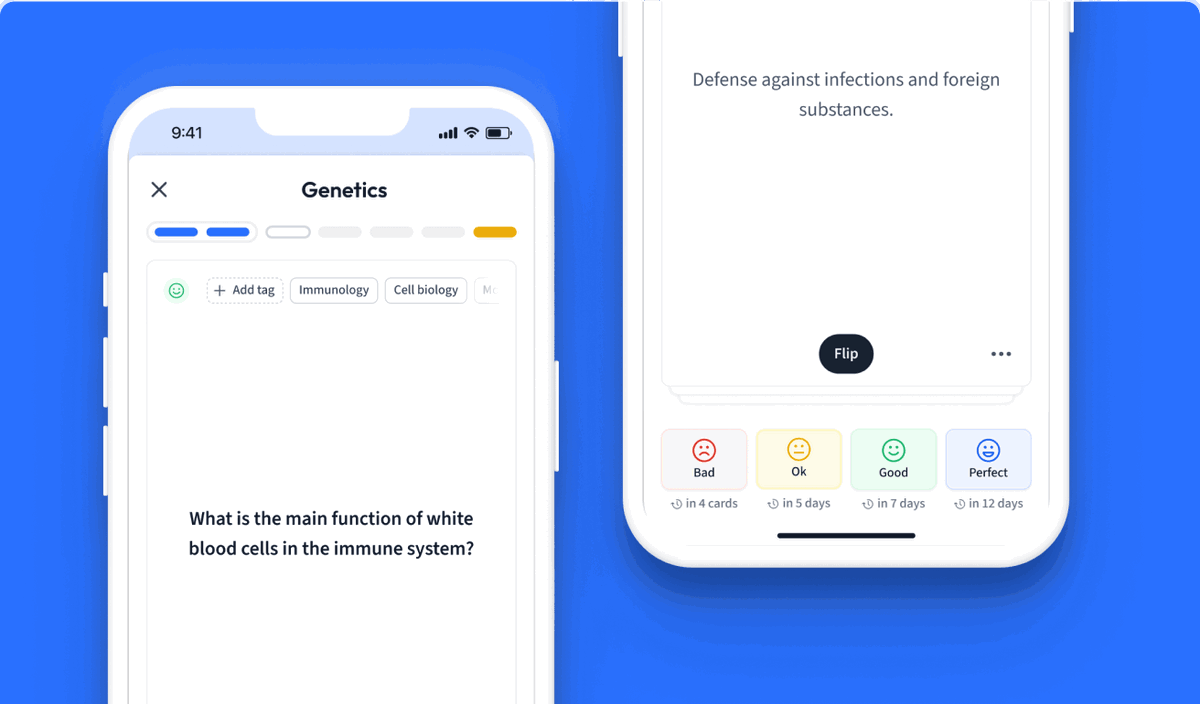
Learn with 266 Gene Technology flashcards in the free StudySmarter app
We have 14,000 flashcards about Dynamic Landscapes.
Already have an account? Log in
Frequently Asked Questions about Gene Technology
What are some examples of genetic technology?
Genetic technologies in areas of stem cells, cloning and gene therapy. It can also be used to create plant GMOs, such as soybean and corn, resistant to pesticides and herbicides.
What is gene technology used for?
Gene technology is used to transfer a gene of interest, created in the lab or isolated from an organism to another organism.
What is recombinant DNA and how is it used?
Recombinant DNA technology combines two DNA molecules from different origins together. This technology introduces genes of value to organisms and creates GMO applications in medicine, science, agriculture, and industry.
What are three uses of recombinant DNA?
Recombinant DNA technology has been proven to be beneficial in producing vaccines, producing hormones, such as insulin, for hormone therapies, and creating crops that give a better yield and are resistant to pesticides and herbicides.
How is recombinant DNA formed?
Recombinant DNA is formed by artifically combining two or more DNA molecules. This process involves five steps:
- The gene encoding the desired needs to be isolated, which involves producing DNA fragments that contain the desirable gene.
- The gene then needs to be inserted into a vector. Vector then carries the gene to the recipient cell.
- The vector delivers the gene to the recipient cells. This delivery process is called transformation.
- The recombinant cells that have successfully transformed and now contain the recombinant DNA must be identified. Marker genes, such as genes that confer antibiotic resistance, assist this step.
- Following identification, the recombinant cells are cloned to produce a large population of transgenic cells.
Why is recombinant DNA important?
Recombinant DNA technology has proven helpful in producing vaccines, hormones for hormone therapies, clotting factors for treating haemophilia and creating crops that give a better yield and are resistant to pesticides and herbicides.
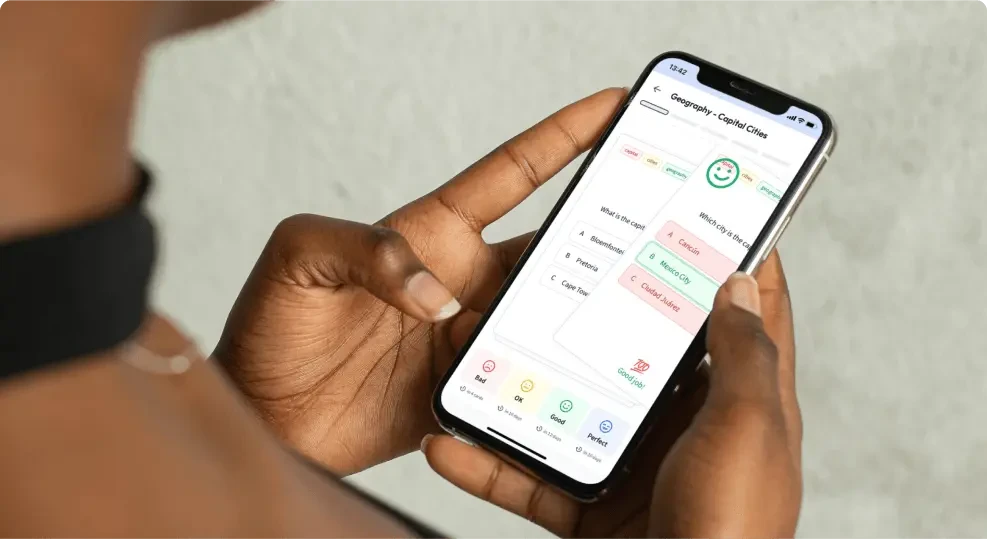
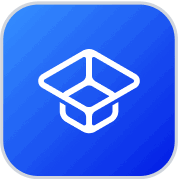
About StudySmarter
StudySmarter is a globally recognized educational technology company, offering a holistic learning platform designed for students of all ages and educational levels. Our platform provides learning support for a wide range of subjects, including STEM, Social Sciences, and Languages and also helps students to successfully master various tests and exams worldwide, such as GCSE, A Level, SAT, ACT, Abitur, and more. We offer an extensive library of learning materials, including interactive flashcards, comprehensive textbook solutions, and detailed explanations. The cutting-edge technology and tools we provide help students create their own learning materials. StudySmarter’s content is not only expert-verified but also regularly updated to ensure accuracy and relevance.
Learn more